Capstone Design
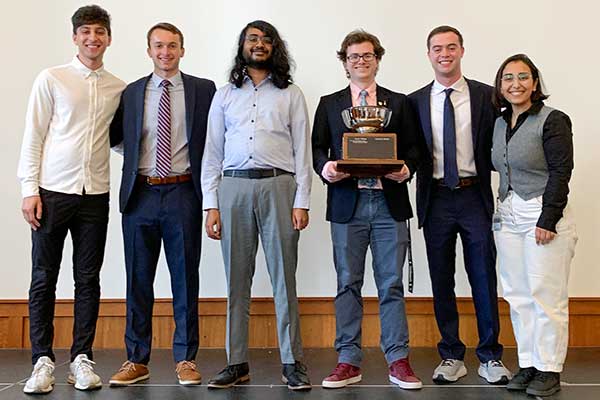
Spring 2024 Bioengineering Capstone Winners – “Scallop Inspired Navigation Device for the Visually Impaired” Team members: Cooper Konz, Joey Maichen, Rahul Mamtora, Pranav Reddy, and Ryan Wilke. Advised by Ronak Ansaripour (pictured on the right)
The Department of Bioengineering has a 2 semester Capstone Design course sequence. Capstone Design 1 takes place during the Summer semesters and focuses on formalization of the design problem and the theoretical aspects of design. Students and faculty work closely together, with individualized guidance and frequent small-group meetings.
Capstone Design offers students an opportunity to apply design principles to create a device or process to solve a relevant bioengineering problem. Continuing with Capstone Design 2 in the Fall or Spring, teams develop, construct, and evaluate prototypes under real-world fiscal, regulatory, and safety conditions. Capstone Design Projects provide deliverables that emulate real engineering practice, with a series of oral presentations and a design history document that is reviewed throughout the course. Students’ efforts culminate in a working prototype, process, or simulation, supported by state-of-the-art facilities on campus.
Quick Facts
- From Fall 2018 to Spring 2022, 60 completed projects
- Estimated $45,000 of sponsored funds to support projects
- 34 Northeastern faculty sponsors from department, college and university
- 26 externally funded projects through industry
Winning Capstone Design Projects
Cooper Konz, Joey Maichen, Rahul Mamtora, Pranav Reddy, and Ryan Wilke Advised by Ronak Ansaripour (pictured on the right)
This device was inspired by the need for navigational aid for those who are visually impaired. Being visually impaired indicates some sort of reduction in vision, whether it be distance or field of view. This results in less information being processed from their surroundings, and detracts from their ability to navigate unfamiliar environments. In order to improve this ability, Team F has taken inspiration from scallops and their wide 270° field of view to assemble a device that analyzes information from the user’s setting and relays it to the user using haptic and audio feedback. A set of cameras and distance sensors are placed in a dock that attaches to the white cane. Both the distance sensors and cameras are able to identify objects in the path of the user and alert the user of the objects’ distance and what type of object it is. The device’s ability to describe additional features of the user’s environment allows for safer navigation.
Shaun Keating, Colby Langer, Emily Devorsetz, Zachary Chau, Grace Matheson, and Natalie Scanlon
Cryopreservation, the method in which biological material is preserved via cooling and stored at low temperatures, is the essential science behind in vitro fertilization (IVF), organ preservation, and cancer cell therapy. Vitrification is a form of cryopreservation that cools biological material by rapidly dunking it into liquid nitrogen. This preservation technique avoids intracellular ice formation, which can damage cells and reduce their viability. The project sponsor, the Center for Engineering in Medicine and Surgery (CEMS) at MGH, currently uses a manual plunge method to vitrify biological samples. This method requires laboratory operators to attach samples to reverse-action forceps and dunk them by hand into liquid nitrogen. Drawbacks to this method include variability in dunking speed, dunking distance travelled, and an increased risk of liquid nitrogen exposure. The CEMS lab is looking to transition away from this manual method, but current automated vitrification devices are highly specialized and do not fit their needs. This project encompasses the development of a novel automated device that plunges samples consistently, improving the repeatability of vitrification experiments. This device must also be portable, easy to use, inexpensive to manufacture, and safe for operators.
Brendan L’Esperance, Victoria Izsa, Anna Pocquette, Julia Kautz, Evan Lens, and Nikola Kapor (not pictured)
Each year in the U.S., more than 1 million cancer patients receive chemotherapy. High -dose chemotherapy used in the setting of hematopoietic cell transplantation leads to rapid and complete alopecia. Chemotherapy induced alopecia induces profound psychosocial and quality-of-life consequences, resulting in anxiety, depression, a negative body image, lowered self-esteem, and a reduced sense of well-being. Additionally, many populations hold hair as cultural identity and even as a spiritual significance across status or rank in society. This project aims to improve on existing cooling cap solutions and patents in the areas of temperature uniformity, to ensure consistent therapeutic results across the scalp, overall cost, and fit ensuring prevention of chemotherapy-induced alopecia.
Transradial amputations – amputations along the forearm – make up 47% of all upper limb amputations. Myocontrol sockets are a common technology used by those with upper limb amputations because it allows terminal devices (eg. hand, wrist, elbow) to actuate in response to EMG signals on the surface of the amputee’s residual limb. The current timeline for custom upper limb prosthesis creation has amputees receiving their final socket after 3-4 visits with a prosthetist over a 3-6 week period. Roughly 1 in 5 upper limb amputees eventually abandon their myocontrol prosthesis, but rejection rates can be lowered if the prosthesis is implemented within the “Golden Period,” which is within the first month of amputation. Reducing the time between amputation and the start of myocontrol socket practice can be done through the use of a fitting socket that conforms to multiple users. In addition, fitting sockets allow patients to trial myocontrol prior to financial commitment and are highly applicable in clinical research where new technologies need to be tested on a variety of transradial users. Our project’s scope is to design and create an open-source fitting socket that can fit a range of transradial amputees (adjusting in both circumference and overall length), incorporates EMG electrodes for myocontrol, and is able to connect to a range of terminal devices (myoelectric hands) so that it may be used as a realistic proxy for a definitive myocontrol socket.
Chimeric Antigen Receptor (CAR) T cell therapies are a rapidly growing autologous treatment option for many diseases such as cancer and autoimmune diseases. This technology allows us to “teach” the body’s immune system to recognize and kill cancer cells within a patient’s body. After extracting and purifying a patient’s T cells, an essential resident of the immune system, medical professionals can alter the cell’s DNA to express a new protein (the CAR protein) on the cell’s surface. After reinfusing the reprogrammed T cells into the patient’s body, this protein directs the T cell to its target cell enabling the T cell to eliminate the cancer cell. Many biotechnology companies have a high interest in making this therapeutic an available treatment option for patients; however, the current standard for evaluating CAR protein/T cell interactions is an expensive and time-consuming process. Therefore, there is a need for a screening assay that produces accurate results while having the capability of running many samples. This project aims to develop an assay procedure that would decrease the time and cost required to not only determine optimal CAR protein signatures, but also optimize the results of any assay based on cell-cell interactions.
Our team modeled the CAR protein/T cell interaction by using mouse-derived T cells and melanoma (cancer) cells. This experimental design determined which process and parameters would maximize the interaction between these two cell types, allowing us to apply our results to similar processes used for CAR protein evaluation. We tested two fluidic systems (shaker table and microfluidics) and mixed these two cell types under various conditions. We then evaluated the effects these mixing parameters (cell densities and mixing frequency) had on the assay outputs. After collecting sufficient data and analyzing the results with various statistical methods, an optimal cell density and shaking frequency was concluded. We determined that these parameters should be used when performing cell interaction assays, such as CAR protein screens, on a shaker table. These results will not only lend themselves to improving the identification of effective proteins for CAR T cell therapies, but once again improve the results from any assay in the industry which measures cell-cell interactions.
Each year, over 400,000 deaths are caused by preventable surgical complications, making it the third leading cause of death in the United States. Quantifying tissue perfusion, or the rate at which blood is delivered to tissue, is a critical factor in reducing surgical errors, as it provides surgeons with information about the viability of tissue and the structures surrounding a given target area. Activ Surgical, the sponsor company of this project, has developed an imaging system called ActivSight, which leverages laser speckle technology to provide real-time tissue perfusion visualization to surgeons during laparoscopic procedures without the need for exogenous dyes, such as the industry-standard indocyanine green (ICG). ICG, while effective in visualizing tissue perfusion, poses complications such as short half-cycles, allergic reactions, and lengthy preparation times. The ActivSight system currently presents confounded measurements of blood flow velocity due to the presence of tissues and fluids which can obscure blood vessels of interest.
The incorporation of autofluorescence imaging via blue light excitation into the current ActivSight system will correct for confounding factors – such as fat, collagen, and bile – which may interfere with the IR light path to target vessels and diminish the laser speckle signal, resulting in inaccurate perfusion information. Blue light fluorescence will allow for a correction equation to be determined that considers the occluding tissue, which will enable the calculation of true blood flow velocities via corrected speckle signal Existing autofluorescence imaging devices are not compatible with laser-speckle imaging systems, which fail to provide unconfounded flow velocities. To satisfy the unmet need, the team has conducted extensive research into current imaging technologies, blue light autofluorescence, and fiber optics to define design requirements and solutions to incorporate a blue light source into the current ActivSight system. In doing so, we were able to differentiate fluorescent signatures for various tissue mimics as related to the depth of tissue and also collected data for a velocity correction algorithm based on fluorescence as compared to confounded flow rate.
Two of our group members, Meghan and Alison, previously co-oped at Activ and brought this project to Northeastern. We were particularly inspired to pursue this project due to the enormity of the unmet clinical need: preventable surgical complications. Ultimately, we were able to provide a proof-of-concept device and algorithm to Activ to initiate additional development in integrating autofluorescence into their current device, ActivSight.
White canes are used by visually impaired pedestrians to provide safety and navigation assistance. Current devices on the market are used for detecting obstacles and identifying the user as visually impaired. While current white canes are useful, there are many improvements that can be made to improve user experience. Common issues include manual deployment and retraction, limited functionality in certain weather conditions and terrains, and poor durability. Our proposed design is a telescoping cane, that can extend and retract automatically with a button, and a multi-terrain tip that can detect ice.
Harmful algal blooms (HABs) accumulate on the surfaces of bodies of water and disrupt the ecosystem, potentially killing aquatic life as well as producing toxins that can cause irritation of the eyes, skin, and respiratory system in humans. Early detection of HABs can greatly reduce or even eliminate some of their effects, but most current technologies are expensive or time-consuming. A device currently in development, Autonomous Real Time Microbial Scope (ARTiMiS), uses image microscopy to detect microbes in water samples at a much lower cost than existing technologies. The DIANA detection system adapts the ARTiMiS to be field-deployable for in situ water quality monitoring, resulting in a low-cost, assemble-yourself floating microscope that will make algal bloom information more accessible to researchers and the general public, providing more data and creating a positive impact on ecosystems and public health.
In this project, a novel cellular engineering process was developed to induce rapid collagen production in patient-harvested cells to be used in collagen patches for soft tissue repairs. Genetic modifications were made to human corneal fibroblasts using CRISPR activation to increase the expression of the genes responsible for the transcription (COL1A1 and COL1A2) and translation (TGF-β3) of type I collagen. Results indicate a 90-fold statistically significant increase from the control. The results of these experiments led to the development of a business model for large scale production that can provide patients in need of a collagen patch following soft tissue repair surgery with one at a fraction of the
current market cost.
By effectively targeting the major bottlenecks of collagen synthesis in human fibroblasts, the cellular engineering design process presented here addresses the current production speed limitations and immunogenicity concerns that currently handicap the use of collagen patches for use in human ligament and tendon repair surgery. By addressing these limitations, a better source of collagen for soft tissue repair patches will be readily generatable as treatment options for the over 15 million cases of tendon or ligament injuries reported each year.
Human Immunodeficiency Virus (HIV) remains one of the leading causes of death worldwide. Pre-exposure prophylaxis (PrEP) is a preventive drug designed to prohibit the further spread and infection of HIV. Truvada is the first FDA approved PrEP regimen for HIV and consists of two drugs, Tenofovir Disoproxil Fumarate (TDF) and Emtricitabine (FTC). When taken daily, PrEP has been seen to reduce HIV infection up to 99%, yet medication adherence remains a challenge on a global scale. To encourage greater adherence, UrSure, Inc. has developed a urine-based lateral flow immunoassay (LFIA) to measure levels of tenofovir (TFV), a metabolite of TDF. However, TFV has a half-life of 17 hours in urine, and therefore, the test can only measure short-term adherence. Conversely, tenofovir-diphosphate (TFV-DP) has a half-life of 17.1 days in red blood cells (RBCs) and can measure adherence up to one month. Our team has designed a blood separation and lysing system to isolate out TFV-DP in RBCs that will be tested downstream on UrSure’s LFIA technology. Our final system consists of four components: a membrane separation filter, absorbent pad,
wash buffer, and lysis buffer. The separation and lysing process is designed to be inexpensive, fast, and composed of widely available materials. Overall, this system will measure long-term measurement of HIV PrEP adherence.
The Sridhar Lab at Northeastern has developed a nanoliposome formulation for the delivery of Talazoparib, a PARP-inhibition drug for the treatment of cancer. This injectable product, NanoTalazoparib (NanoTLZ), enables superior bioavailability and efficacy compared to the orally administered drug. The lab currently produces NanoTLZ using a commercially available microfluidic chip system that mixes an organic stream containing the drug and liposome components with an aqueous stream to facilitate nanoliposome self-assembly. The current system is expensive and low throughput, and the lab seeks proof-of concept for a device fabricated in-house to address its limitations. Harnessing the laser cut and assemble fabrication method pioneered by the Koppes Lab, we have created a low-cost, higher throughput microfluidic chip to produce NanoTLZ.
Microbes are ever-present in water distribution systems, and pathogenic species pose an ongoing threat to human health. Current techniques for water quality monitoring are slow and labor-intensive, resulting in a time lag between contamination and detection. There exists a significant gap for a low-cost, precise, rapid, and automated method of monitoring microbial concentrations in drinking water. To meet this need, we aim to develop an imaging device that detects and quantifies microorganisms in drinking water systems. Our design features carefully controlled, automated fluid intake and disposal, a modular optical configuration capable of resolving objects under 2 microns, and a machine learning classification algorithm capable of discerning contaminants in images. The integration of these design solutions creates a device capable of meeting global needs for water monitoring.