BioE’s Bajpayee Awarded NSF CAREER Grant for Drug Delivery Biomaterials
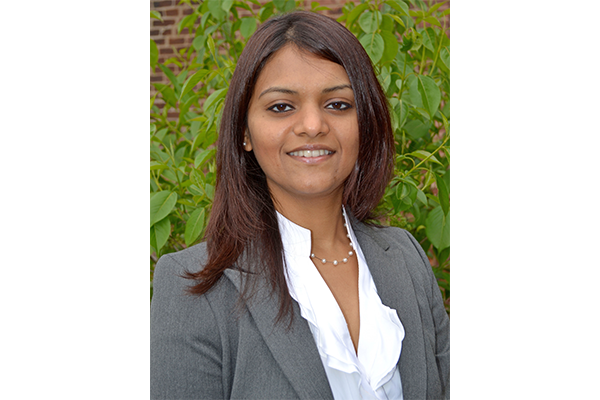
BioE Assistant Professor Ambika Bajpayee received a $630K NSF CAREER Award for “Developing electrically charged biomaterials for targeted drug delivery to negatively charged complex tissue environments.” Her research will investigate how to improve drug delivery in tissues within the human body that are not receptive to systemic or local drug delivery methods due to their high negative charge density and lack of blood vessels by using charge interactions.
Ambika Bajpayee, assistant professor, bioengineering (BioE), has been awarded a $630,000 National Science Foundation (NSF) CAREER grant to investigate how to improve drug delivery in dense, avascular human tissues like joints, cartilage, and tendons.
“These kinds of tissues in the human body are very difficult to target with traditional drug delivery methods such as oral medications or injections because they are so dense and have few blood vessels,” says Bajpayee. “We are working on a local, targeted delivery process that would allow drugs to reach these areas, which could vastly improve treatment for osteoarthritis and other musculoskeletal diseases.”
Because these tissues are negatively charged, Bajpayee is creating positively charged biomaterials that are drawn to the tissues’ cellular matrix.
The impact of modifying different physical and chemical properties of positively charged biomaterials on their transport will be evaluated using cartilage and synovial fluid, as a model of negatively charged tissue environment.
Bajpayee’s research is using bovine knee joints and the surrounding synovial fluid—which is an integral part of joint function in that it delivers critical lubrication and nutrients, but it can also be a barrier to drugs reaching the joint where they are needed most.
In order to successfully bypass binding with the synovial fluid—which is also negatively charged—and get to the joint itself, Bajpayee and her team are working with weaker charge interactions instead of very strong binding mechanisms for specific receptors on individual cells.
“The main challenge in drug delivery for these dense tissues is that drugs typically ‘stick’ to whatever they find first and can’t penetrate deeper,” says Bajpayee. “That’s why we like charge interactions—because of their weak and reversible binding nature, they can bind and unbind and move forward, so the drugs can make their way deep into the tissue to reach cells close to the bone.”
The study will reveal key structure-property relationships in electrically charged biomaterials and complex negatively charged musculoskeletal tissue environments in both healthy and diseased states that can be generalized and tuned to target other charged tissue systems. Long-term, the program has wide applicability as it can be extended to other tissues with similar properties (meniscus, intervertebral disc, fracture callus, eye, mucus), diseases and for delivery of various drugs and imaging agents – ultimately facilitating their clinical translation.
Part of the goal of NSF CAREER grants is to highlight faculty who serve as academic role models in research and education and lead advances in the mission of their department or organization. For Bajpayee, she intends to work toward patenting this technology to get it out into the marketplace.
“We aim to utilize Boston’s ecosystem of startups, pharmaceutical companies, biomedical firms, venture capitalists, and more to get this novel drug delivery system out into the world,” says Bajpayee.
Bajpayee hopes to establish a yearly collaborative consortium where these industries can come together to help undergraduate and graduate students think about different ways to translate their scientific work.
Also through the grant, Bajpayee is in the process of creating an exchange program for undergraduate students from universities on the East and West coasts and those in the center of the U.S. with the greater goals of academic and cultural exchange.
Abstract Source: NSF
This proposal investigates how physicochemical properties of polyvalent cationic macromolecules affect their electro-diffusive transport within negatively charged tissues and their cellular microenvironments and uses this knowledge to rationally design cationic carriers for targeted drug delivery to tissues based on their negative fixed charge density (FCD). Human body contains several negatively charged tissues that are inaccessible to both systemic and local drug delivery due to their avascular, dense extracellular matrix. This high negative FCD, however, can be converted to an opportunity by modifying therapeutics to add optimally charged cationic domains such that electrostatic interactions can enhance their transport rather than hindering them. This long-range, weak-reversible charge-based intra-tissue binding can be synergistically stabilized by short-range binding effects (H-bond, hydrophobicity) such that these carriers can have long residence times even in degenerated tissues with diminished FCD. Using cartilage as a model target tissue of high negative FCD owing to its high density of glycosaminoglycans (GAGs), this proposal will investigate the effects of physicochemical properties of cationic carriers on their transport in aim 1. Cationic peptide carriers (CPCs) with the same short length but varying net charge comprising of arginine (exhibits H-bonds and charge interactions) or lysine (primarily charge) will be designed to determine an optimal configuration for fastest intra-tissue diffusivity, full-depth penetration, highest equilibrium uptake, and long-term binding to target chondrocytes residing in both healthy and arthritic cartilage. In addition to short intra-joint residence time of drugs due to rapid exit from lymphatics, cartilage targeting is further compromised by competitive binding with synovial fluid, that comprises of both negatively charged hyaluronic acid and hydrophobic globulins. In aim 2, hydrophobic or hydrophilic tails will be added to the optimized CPC designs to investigate the synergistic or competitive effects on charge-based binding within cartilage of varying FCD in presence of synovial fluid. In aim 3, these optimized cationic motifs will be anchored on exosome’s anionic surface in varying densities to demonstrate improved targeting of arthritic cartilage in presence of synovial fluid.